Comparative study of a broad qPCR panel and centrifugal flotation ... - Parasites & Vectors
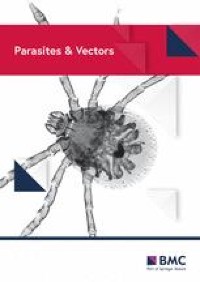
To the authors' knowledge, this represents the first study to compare the performance of ZCF to this commercially available broad-spectrum qPCR panel for intestinal parasite identification in a collection of fecal samples from privately owned dogs and cats. When comparing the overall performance to detect parasite load, frequency of parasite-detected samples, and ability to detect co-infection, qPCR detected a statistically significant greater frequency compared to ZCF. This difference was especially striking when evaluating the number of co-infections, where qPCR identified nearly three times as many. Agreement calculations demonstrate the overall ability to detect parasite burden and parasite-detected samples between ZCF and qPCR to be substantial however also highlights the specific parasites where ZCF had a reduced to absent ability to detect (G. duodenalis, Trichuris spp., Cystoisospora spp., T. blagburni, and T. gondii) where agreement was only slight to fair. These data suggest that while ZCF remains a highly affordable, fast, and widely available GI parasite screening test, molecular diagnostics such as this qPCR panel are poised to undergo consideration as a reference method for parasite detection because of its ability to maintain affordability and rapid turnaround while additioanlly providing the advantage of increased parasite detection.
While commonly used by many veterinary clinics and reference laboratories, the limitations of ZCF for intestinal parasite screening have been demonstrated previously. This method is outperformed by the double centrifugation method and fails to identify smaller parasite structures such as whipworm eggs because of the relatively low density of zinc sulfate [22]. This may be one explanation for ZCF's inability to detect certain parasites compared to qPCR in this study and contributed to a reduction in agreement between the two methods as well as co-infection. However, double centrifugation methods are less time and cost efficient and therefore limit the accessibility of this test. Molecular diagnostics on the other hand have been previously described to provide an advantage in diagnostic sensitivity in multiple applications.
The 'omics' revolution in the field of biological sciences over the past 2 decades has vastly improved access to molecular and genetic resources of parasites. This explosion of research and collaboration has opened doors for the deployment of molecular diagnostic techniques with improved performance [5]. Additionally, advanced molecular tools such as CRISPR technologies are furthering the functional understanding of new mutations correlating with anthelmintic resistance [23]. These molecular techniques have the unique ability to increase sensitivity and specificity of diagnostic tools, enabling the generation of fecal surveillance data, which supports developing new control initiatives for parasitic diseases and alerts us to GI parasites of One Health concern, e.g. Giardia strains with zoonotic potential and A. caninum treatment-resistant hookworms [13, 15].
There is an increasing body of evidence supporting the superiority of molecular tests to detect parasitic infections with higher sensitivity, more breadth, and more functional qualities such as anthelmintic resistance and zoonotic variations [9, 23,24,25,26,27,28,29]. Conventional fecal diagnostic methods, like centrifugal flotation and antigen detection, are solely based on morphological features or secretory/excretory antigens [30]. These methods also lack the ability to read into the functional differences of parasites or detect antimicrobial treatment resistance mechanisms or differences in parasite zoonotic or non-zoonotic potential. In addition, the morphological assessment of parasitic eggs or cysts, in particular for Giardia, often cannot be identified or differentiated by ZCF in a general practitioner setting [31]. Similarly, biological structures such as pollen grains, plant and yeast cells, or fungal elements may be misidentified as parasitic structures by fecal centrifugal flotation techniques [32], while variations in egg size due to sample age, temperature, humidity, and even anthelmintic therapy can make accurate fecal microscopy more difficult [33, 34]. These factors can lead to a relative lack of specificity, which may impact the overall sensitivity of fecal ZCF.
In molecular diagnostics, Sanger sequencing can be used to confirm the presence or absence of parasites when conventional tests are negative. This provides an agile platform for sample characterization and subsequent assay optimization. In our study, these protocols were implemented to confirm discordant roundworm-detected samples, hookworm resistance marker-detected samples, as well as the Giardia strains with zoonotic potential. Further confirmatory testing was performed for a selection of hookworm samples, 12 whipworm samples, and two of the 36 Cystoisospora samples. Additionally, of the 134 qPCR-detected Giardia duodenalis samples which were ZCF negative, we selected six samples, all of which were confirmed by qPCR as G. duodenalis. Based on the unique ability to prove the existence of nucleic acid for particular parasites by Sanger sequencing using outside primers, the specificity of molecular tests can be adjusted in most qPCR applications to approach 99%, as described in our work.
Fecal qPCR, like all biological tests, is not perfect, and potential for false positives and negatives remains. In our study we observed a low frequency of discordant results, some of which represented ZCF-positive but qPCR-undetected samples. The example in this study relates to those roundworm samples where Toxocara was identified by ZCF but undetected by qPCR. One explanation relates to the potential subjectivity of microscopy and its potential for misidentification of plant, yeast, or fungal material as GI parasite structures. However, another explanation, and as shown with our novel roundworm species findings, morphologically clearly identifiable Toxocara eggs can be observed with fecal ZCF but are reported undetected by molecular methods. As in this study, the phenomenon can be secondary to the existence of novel parasite genotypes which are not detected by fecal qPCR because of mismatches on the primers and/or probes of a given qPCR test. Characterization of new genotypes, therefore, is of great use in molecular diagnostics to minimize false-negative results and further elevate molecular diagnostics to the reference method status. In our study, nine of the 16 ZCF-positive roundworms were confirmed as T. cati with unique sequence variations rendering the qPCR test undetected. Updating the qPCR primers, and if necessary hydrolysis probe sequences, to include those novel strains into the roundworm specificity definition is a necessary and elegant way to expand the performance of the Toxocara spp. qPCR. Existence of distinct species-adapted genotypes as a result of biological adaption to their respective host has been described for other parasites, such as the separation of the tapeworm Dipylidium caninum into a feline and a canine genotype [35].
Limitations of this study include those inherent to retrospective study design including a lack of clinical data regarding presenting signs, medication history, reason for submission, etc. Given the time of year and region where the samples were collected, it is likely that the parasite detection frequency results should not be extrapolated or interpreted as representative of prevalence as that was outside the scope of this study. For example, the age, region, and season could have biased this population toward a greater number of roundworm infections as has been described previously in North America [1, 36]. Another limitation of this study is the lack of corresponding double-centrifugation results for this collection of samples to provide a reference method for comparison. While ideal, double centrifugation is not representative of intestinal parasite screening for veterinary patients from a commercial laboratory, and the authors of this study opted instead to compare two tests readily available to most clinicians. Future studies would be necessary to compare qPCR and double centrifugation using different specific gravities of sugar and salt solutions to determine their diagnostic concordance.
As mentioned above, roundworms comprised the largest proportion of parasite-detected samples. This provided the opportunity to investigate the presence of previously unknown roundworm nucleotide regions and variants. Diligence in the selection of nucleotide regions for the design of qPCR tests is of upmost importance. This is shown in our work through the roundworm example, where unknown genotypes or subtypes of particular nematode species can impact the sensitivity of molecular methods. However, due to molecular advancements in identifying previously unknown nucleotide sequences through conventional Sanger sequencing or any of the newer deep sequencing technologies, qPCR tests can be evolved and improved to detect novel sequence variations. As evidenced with the updated roundworm qPCR test, a diagnostic sensitivity of > 99% can be achieved when sequence mismatches on primers and probes are eliminated. This allows the detection of strains of clinical veterinary relevance with the highest analytical and diagnostic accuracy. Furthermore, using outside sequencing primers can not only discover new parasite genotypes but also confirm discrepant positive qPCR results. Additionally, the species-specific identification of T. cati in dogs likely indicates GI parasite passage, rather than true infection, and type of finding provides valuable information for the practitioner. Taken together, new genetic information can be used to further innovate and fine-tune molecular tests to detect previously undetected genotypes and is a key aspect of bringing molecular testing to the forefront of endoparasite surveillance and wellness screening in veterinary medicine.
Comments
Post a Comment